Edge of the atmosphere - what, where, and who cares?
- Israel Silber
- Jan 31, 2017
- 8 min read
Wearing the right glasses
In our everyday life we tend to think that when we fly from place to place inside jet airplanes 10 km above the Earth's surface, we effectively touch the edge of the atmosphere. Our comprehension is that the International Space Station (ISS) and polar orbiting satellites are looking at us from space and that a man has insanely jumped from space. But where exactly is that separating line between the planet's atmosphere and the cold reaches of space located? Is there such a distinct line at all? Well, to answer these questions, first of all, it is important to know what glasses are we wearing while we examine the atmosphere? more precisely, in what aspects of the atmosphere are we focusing on, and for what purpose?
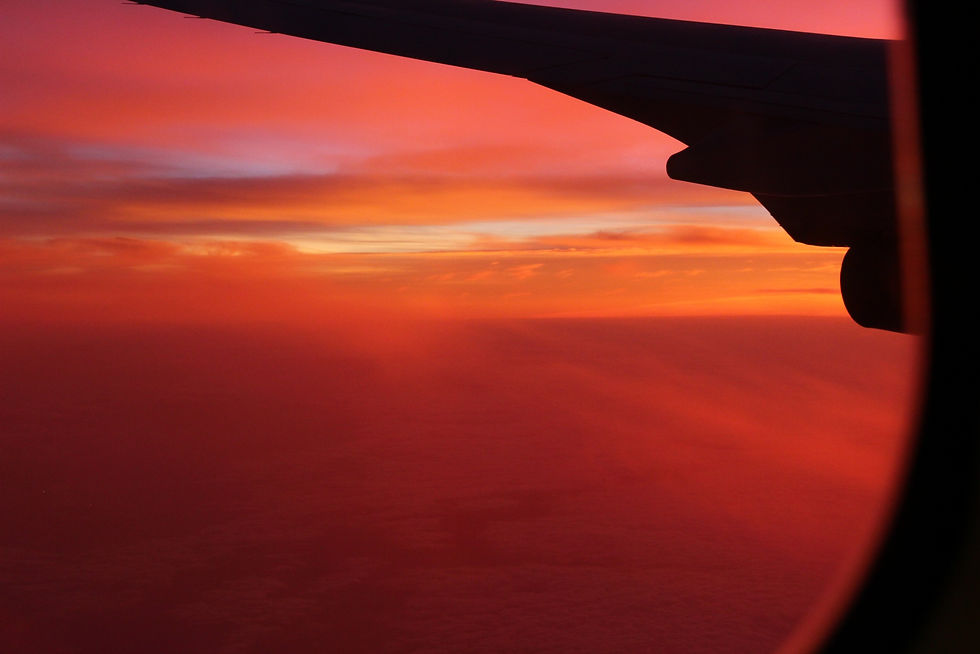
There are four main pairs of "glasses" used to observe the atmosphere; the temperature profile glasses, the composition glasses, the ionization glasses, and the gaseous escape glasses. The temperature profile glasses examine the atmosphere based on the temperature trend as a function of altitude, also known as the lapse rate. Our general everyday conception is that as we ascend (e.g., while climbing a mountain, or flying in an airplane) the temperatures drop. That is true, but only because we live in the troposphere, the lowermost layer of the atmosphere as seen while wearing the temperature glasses. Within the troposphere, the temperature drops with altitude, but at a certain point (known as the tropopause), which is located somewhere between ~8 km (at the poles) and ~15 km (at the equator), the temperature changes its trend and starts to rise with altitude. This is where the stratosphere is located (and the celebrity ozone layer, which is the source of this warning with altitude). Above the stratosphere is the stratopause, then the mesosphere (where the temperature drops once again), and above it lies the thermosphere, where the temperatures rise to a few thousand degrees centigrade. In between the mesosphere and the thermosphere sits the mesopause, which is known as the region of the atmospheric profile with the lowermost temperatures. This thin layer of the atmosphere can often be easily detected in photos taken from the ISS by the narrow and pale greenish and reddish stripes known as the "airglow" (sometimes also referred to as "nightglow"), which is typically located within the mesopause.
The composition glasses are used to divided the atmosphere into two main layers; the homosphere and the heterosphere, separated by the boundary known as the turbopause (at ~100 km). The homosphere is the lowermost part of the atmosphere where the air is well mixed and is essentially what we call air, that is 78% nitrogen, 21% oxygen, 1% argon, and traces of carbon dioxide and other gases. However, within the heterosphere, the various gases of the atmosphere are no longer well mixed, but separated into sub-layers, based on their molecular mass. We can easily understand this concept by taking a glass of water and pouring some high-quality extra virgin olive oil into the glass (every other oil would work as well, but I am a fan of olive oil, so it is hard for me to hide it). When we properly blend the oil and the water, we get sort of a nice mixture. Now, letting the mixture rest, physics come into play; as water are denser than oil (i.e., have a higher molecular mass) the gravity force differentiate the water and oil into layers, where the heavier water is beneath the light oil. That is the exact same process happening in the heterosphere; there is almost no mixing, so gravity can take its toll.
The ionization glasses are generally rather simple to separate; if the air is ionized (i.e., even a tiny fraction of the air particles are electrically charged), you are within the ionosphere. The ionosphere, which is mainly generated and maintained by highly energetic solar radiation, lies from ~60 km up to ~1000 km, and although this general separation sounds very simple, this region is highly complex and is separated into sub-regions; the D-region, the E-region, the F1-region, and the F2-region. These odd names are just old terms which were adopted to honor science history. The E-region was the first ionospheric layer to be detected (and theoretically speculated) at the beginning of the 20th century, and was named that way so that the "E" resembles "electrified". When the other ionospheric layers were detected (each with its very unique physics and chemistry), they were termed in a simple alphabetical order (the same principle was applied for the F1 and F2 layers at a later stage).
Finally, there are the gaseous escape glasses. The general separation here is relatively simple as with wearing the ionization glasses; the region where individual atoms can escape from Earth's gravitational attraction is known as the exosphere. That is possible as the the force of gravity weakens as we move further away from the center of the Earth, but more importantly, the air density is so low, that a single atom traveling upwards will not experience on average more than one collision with another atom before it escapes into space. Physicists treat the atmosphere as a compressible fluid, thus relating the pressure, density, and temperature of the atmosphere to each other in what is known as the gas law. Nevertheless, that is no longer possible within the exosphere. The exosphere is generally located at ~500 km. Below it is the barosphere, and the boundary between these two regions is known as the exobase.
Vague boundary
We just met the four main glasses to observe the atmosphere, but nowhere was the edge of the atmosphere mentioned. Why is that? The answer to this question is that there is no such distinct boundary. The atmosphere essentially fades into space, However, people tend to determine the edge of the atmosphere based on the observing glasses they wear and the purposes for which they want to mark the edge of the atmosphere. Some scientists might mark this boundary right at the exobase, while others may determine a specific altitude as the line which above it, you are in space. This partitioning or the adoption of one can also emerge from commercial reasons as well, in order to boost sales.
We now understand that the determination of the edge of the atmosphere is dependent on people's incentives, but as a matter of fact, and as with almost every atmospheric aspect, the picture becomes even more complicated.
The mishmash problem
Multifocal glasses are a good solution for people with vision impairment. They allow these people to see things that are closer to them, as well as the distant objects on the horizon. Because of these glasses, a full picture of the surrounding world can be achieved.
The different aspects of the Earth's atmosphere are somewhat similar; we have to use a multi-focal observing glasses, in order to attain a broad understanding of the atmospheric behavior and processes governing it. That said, even the determination of the altitudes of the different layers and boundaries mentioned above cannot be made without the consideration of altitude of other layers in other aspects, for example, the ion concentrations of the ionospheric layers is dependent to some degree on the atmosphere's temperature. Vice versa, the ionization processes can warm the surrounding atmosphere and are effectively the heating source of the thermosphere. All the processes and interactions between different aspects of the atmosphere are very well arranged and do not leave anything to chance. Nevertheless, the fact that we do not understand all of these processes and do not know all the participating parameters and the extent of their influence, makes these interactions look like a giant mishmash. Hence, it is not coincidental that many data sources incorrectly mix different atmospheric attributes, especially when focusing on the murky upper atmosphere.
Unraveling the mess in the attic
In order to gain a better understanding of the atmospheric dynamics, chemistry, and physics, this entanglement has to be resolved. This objective challenges the atmospheric science community, which tries to illuminate new pieces of information, often in infinitesimal increments at a time. That is performed by analyzing and interpreting atmospheric observations using different "pairs of glasses", and nudging the results in different types of computer models.
This methodology of acquiring more information and better insights about our planet's atmosphere by using observations and modeling works pretty well, although in almost every field of research, there are plenty of hurdles on the way to thorough knowledge (otherwise, they would not be areas of research, because everything is already known, right?). The upper part of the atmosphere for example (we consider it from ~60 km, at the base of the ionosphere, up to several hundred kilometers), poses many such hurdles. Similar to atmospheric cloud measurements in Antarctica, a large portion of these hurdles results from a lack of observations, which is really not a coincidence, especially at about 60-95 km above the Earth's surface, within the upper mesosphere-lower-thermosphere (MLT) layers (using the temperature profile glasses) and the D-region of the ionosphere (using the ionization glasses). This region is too high for weather balloons (which normally ascend up to ~40 km), too low for in-situ measurements by polar-orbiting satellites (which typically have orbiting altitude above 300 km), and too variable for rocket experiments, which are spatially and temporally limited (although they still provide valuable information).
However, knowledge about this intriguing part of the atmosphere is essential; This part of the atmosphere "communicates" with the lower part of the atmosphere (mainly through different types of pressure wave, which to some extent are similar to sound waves, only at much lower frequencies, far below our hearing range). In addition, satellite trajectories are affected by atmospheric drag, which requires orbit corrections to be made. As mentioned above, the atmosphere fades into space, but some particles do exist within satellite orbit altitudes, and they are more than enough to exert drag, which is crucial on the long term. In addition to satellite drag, one of the main areas affected by processes in the upper atmosphere is the propagation of electromagnetic waves, which we naively employ in our everyday lives (e.g., GPS, satellite TV, radio, etc.). The characteristics of wave propagation are highly dependent on the atmospheric properties. For example, GPS geolocation, which is based on reception of signals emitted by a constellation of satellites, at high frequencies, within the microwave range (yup, same as our microwave ovens), is extremely sensitive to changes in the atmospheric temperature and ionization profiles. Both of these parameters may change the diffraction patterns of the emitted signals traveling from the satellites to our GPS receivers (often located in our cell phones). This may result in a lower accuracy of our receivers, and ultimately, in us arriving late for a meeting or a date, just because we did not examine the actual route to our destination, instead of relying on the GPS "magic". Waves at lower frequencies (below the UHF frequencies) are manipulated for TV and radio reception and are also exploited for ground communication within several military bodies. All of these uses are made possible because waves at these low frequencies are reflected off the ionosphere due to different processes. The thumb rule here is that the lower the frequency, the lower the reflection height. Nevertheless, the properties of the received signals, i.e., their phase and amplitude, are sensitive to disturbances in the reflection region charged and neutral atmospheric constitutes' conditions, e.g., concentrations, temperatures, mixing, etc.
Therefore, the different oscillations, perturbations, and general changes in the upper atmosphere are important to characterize, comprehend, and monitor. Understanding these processes allow geolocation calculations with higher precision and proper reception of radio waves. The extreme cooling of this part of the atmosphere due to increased greenhouse gases' emissions, which is one order of magnitude stronger than the lower atmospheric warming we experience down here, demands that these monitoring and characterization efforts will be made at large time scales as well, on the order of decades. As in-situ measurements are not a widespread option to gain new insights about the upper atmosphere, remote-sensing instruments are commonly used to acquire upper atmospheric observations, and consequently expanding our knowledge. These instruments are situated both at the ground and on satellites, although the satellites used to observe the upper atmosphere can be counted using one hand. During my PhD studies, I used a few remote sensing instruments (both ground-based and space-borne) to monitor the MLT, the ionospheric D-region, and the interaction between them. This was a bi-focal glasses effort, which allowed me to gain several of these infinitesimal increments of knowledge about this intriguing part of the atmosphere.
Undoubtedly, plenty of work should still be made to achieve some order in the comprehension of the upper atmosphere, the atmosphere's "attic", but it is worth it, especially for those who are still on the hunt for the location of the edge of the atmosphere.